Central to the EV revolution is the EV battery – a highly complex and intriguing piece of technology that delivers power for our electric cars. In this comprehensive guide, let’s unveil the mystery of EV batteries by analyzing their construction, the minerals that are in use, and the factors shaping this fast-developing industry.
The Power Behind the Plug: Understanding EV Battery Chemistry
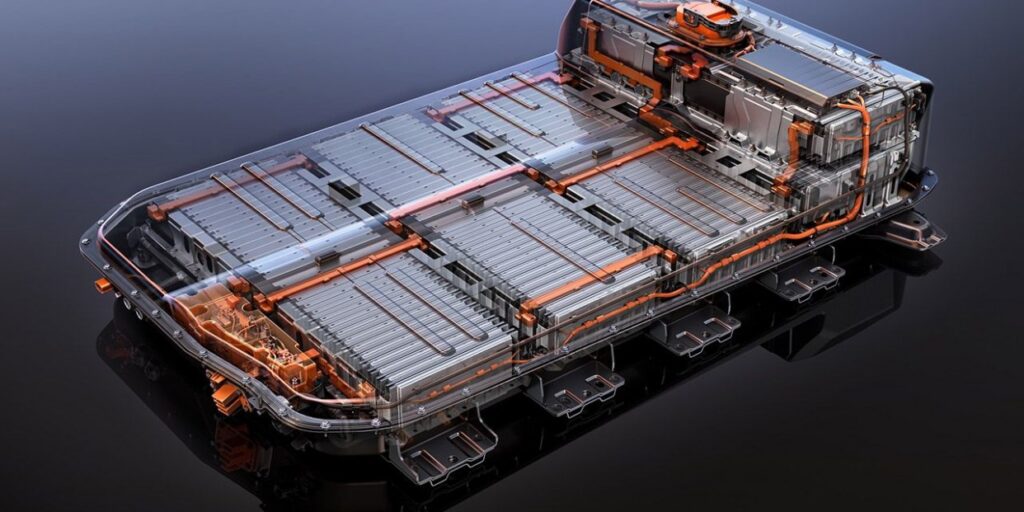
Img Credit- arenaev.com
Many electric cars are powered by lithium-ion batteries that are well known for being very efficient and long-lasting. They are particular to electrical vehicles because of their high energy density, resistance to multiple charge cycles, and low self-discharge rate, ensuring minimal energy loss when not in use.
Within the domain of lithium-ion batteries, two dominant chemistries have emerged: lithium nickel manganese cobalt oxide (NMC) and lithium iron phosphate (LFP). All of them come with a range of advantages and disadvantages.
NMC Batteries:
Higher energy density, thus increasing their mileages.
High performance under cold conditions.
Use more expensive metals such as cobalt, nickel and manganese.
Shorter lifespan among LFP.
LFP Batteries:
The thermal energy of hydrogen is lower but it has high enthalpy.
Tolerate high temperatures better
Make use of materials that are readily available and plentiful such as iron and lithium
Longer life span with higher lithium needed.
Cheaper, though seldom reclaimed.
The NMC and LFP batteries are chosen after taking into consideration the factors like the desired range, operating conditions, and cost aspects where there is no universal “best” choice for all EVs.
Bringing to Light the Secrets of Lithium-Ion Batteries
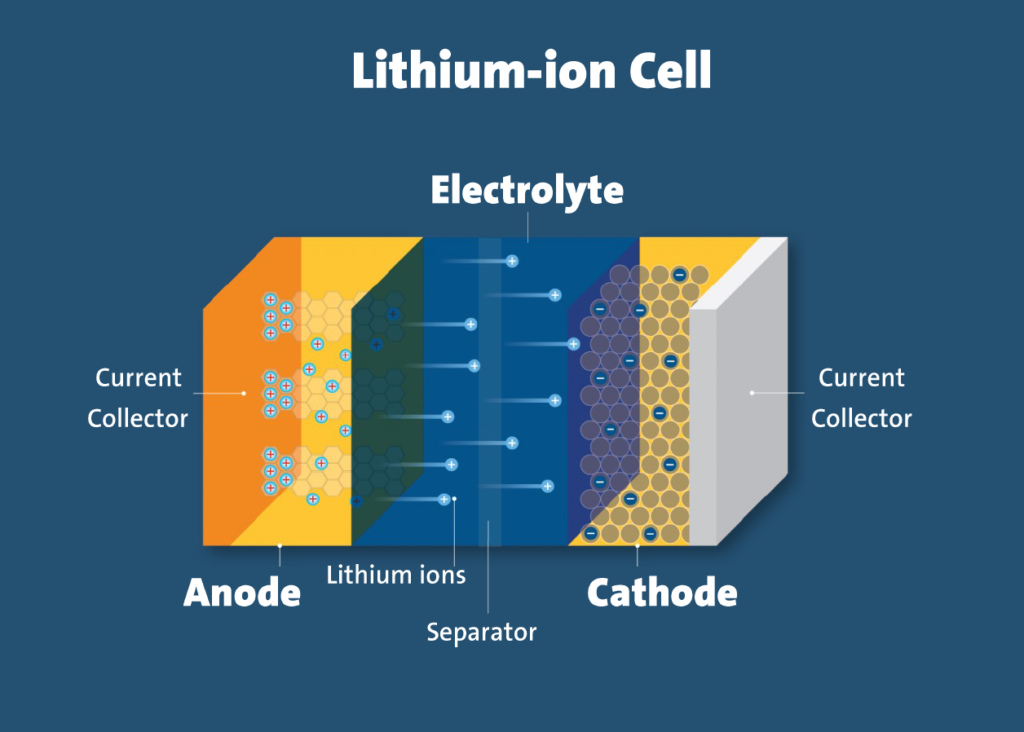
Img Credit- ul.org
An important subject for the understanding of EV operation is how these batteries work. At the core of a lithium-ion battery lies a delicate dance between two electrodes: the anode (negative) and the cathode (positive).
At discharge, the lithium-ion ions migrate from the anode to the cathode through an electrolyte bridge, generating a current that supplies power to the vehicle. When charging, ions move in the opposite way back to the anode in order to become available for the next discharge cycle.
The ion exchange takes place through a thin, porous separator that recovers only lithium ions whereas prevents direct electronic short circuits. This amazing ballet of electrons and ions is what synergizes chemical energy into the electrical power that is used by EVs to propel forward.
The Critical Mineral Equation: Sourcing the Building Blocks
Although lithium-ion batteries are an engineering milestone, however, they are still dependent on a few important minerals whose trading circuit is less simple. Cobalt, lithium, manganese, nickel, and graphite are among the important components, and the ways their extraction and processing are done have raised questions of ethical mining, human rights, and environmental damages.
The employment of children in mines, the unavailability of workers’ safety measures, and the damage of local communities and sceneries are some of the issues that many mining companies experienced. With the growing need for these minerals, the responsible extraction, recycling, and use of minerals is thus an urgency issue to be looked into.
Conclusion
As the electric vehicle revolution adds up momentum, the search for better, more efficient, and more environmentally friendly EV batteries never stops. Researchers and producers are investigating new chemistries other than solid-state batteries which are providing already high energy density, improved safety, and others.
Also, attempts are made to set up resilient recycling programs and closed-loop supply chains, which will be functioning in such a way that the valuable materials will be recovered and reused, thereby doing away with the adverse effects of mining and processing the materials.
Through the elimination of myths surrounding EV batteries and comprehension of the interconnectedness of chemistry, materials, and ecology we will be able to shape decisions that move the rotation forward and at the same time contain the environmental footprint. As customers, investors as well as government representatives, we as a whole will determine the future of this revolutionary technology and help on that path to a cleaner, brighter, and more sustainable world.
FAQs
Q1: What types of batteries do most electric vehicles use?
A: Majority of the electric vehicles are run by lithium-ion batteries and the two most widely used battery chemistries are lithium nickel manganese cobalt oxide (NMC) and lithium iron phosphate (LFP).
Q2: What are the key advantages of lithium-ion batteries for EVs?
A: Lithium-ion batteries have extensive energy capacities to store a lot of power, can withstand several charge cycles, have low self-discharge rates, and their reliability is maintained across a wide range of temperatures.
Q3: What is the difference between NMC and LFP batteries?
A: NMC batteries have a higher energy density that results in a longer range but cost more and have a shorter life span. LFP batteries may replace energy density with thermal stability, use more abundant materials like metals, cost less, and last longer.
Q4: How do lithium-ion batteries work?
A: Lithium-ion batteries work by the flow of lithium ions in between the anode (negative electrode) and cathode (positive electrode) passing through an electrolyte medium.
Q5: What are some ethical and environmental concerns around EV battery materials?
A: Mining for various minerals of batteries has raised several challenges such as human rights abuses, child labor, safety issues of the workers, and environmental degradation which requires responsible sourcing.
Amit Gupta is an extreme environmental enthusiast and a passionate EV blogger who completed his B.Tech in Computer Science. He explores the emerging environmental technologies and discoveries in EV sector, sharing his valuable insights and tips on his blog which are based on his personal experiences. Through his articles, Amit Gupta aims to simplify the complex EV sector concepts and empowers his readers to make accurate decisions in our rapidly changing world. Follow him on Facebook and linkedin to stay updated on his latest posts.